Introduction
Materials and Methods
Plant material
Firmness, fresh weight loss and respiration rate
Specific gravity (SG), dry matter (DM) and starch content
Extraction and quantification of glycoalkaloids
Chipping of the tubers
Extraction and quantification acrylamide from the chips
Determination of color of chips
Overall sensory evaluation
Statistical analysis
Results and Discussion
Firmness, weight loss and respiration rate
Specific gravity, dry matter, and starch content
α-solanine and α-chaconine and total glycoalkaloids
Acrylamide content, color changes and sensory evaluation of the potato chips
Conclusions
Introduction
Potato (Solanum tuberosum L.) is a solanaceous starchy crop that has highly diverse landraces with a variety of tuber shapes and colors that distributed and grown worldwide (Friedman, 2006; Machida-Hirano, 2015). Potato is the world's fourth-largest food crop, following maize (corn), wheat, and rice with a worldwide production of 376.82 million tons from 19.2 million ha valued at $111.06 billion (FAOSTAT, 2016). China leads the global potato production by 96.96 million tons, followed by India and Russian Federation, which produced 45.89 and 32.38 million tons, respectively, in 2016 (FAOSTAT, 2016). According to FAOSTAT (2016), potato production in the Republic of Korea was 631,596 tons from 24,041 ha in 2016, which was valued at $121.66 million.
Sustainable production of potato is vital for ensuring long-term food security due to its high productivity and generation of more food per unit area and per unit time than maize, rice, and wheat (López et al., 2013). About 80% of raw potato tubers is water, and the remaining 20% is dry matter, starch make up about 60-80% of the dry matter. Potato tubers are also rich in calcium, potassium, vitamin C, and protein with proper amino acid balance. Moreover, tubers supply high levels of energy ascribable to their rich starch content (FAO, 2008).
Glycoalkaloids are biologically active secondary metabolites that usually found in plants of the genus Solanum. Solanum tuberosum contains a significant amount of α-solanine and α-chaconine, which make up 95% of the total glycoalkaloid content (Shen et al., 2014; Karaboğa Arslan and Yerer, 2018). The natural function of glycoalkaloids is probably to serve for protection against the attack insect pests and microorganisms (Cantwell, 1996). Glycoalkaloid levels can be affected by cultivar’s difference and could be influenced by postharvest environmental factors such as light, mechanical injury, and storage (Friedman, 2006). Potato tubers which contain more than 200 mg kg-1 total glycoalkaloids of fresh tuber weight have a bitter off-flavor and may cause gastro-enteric symptoms, coma, and even death (Coxon et al., 1979; Cantwell, 1996; Sotelo and Serrano, 2000; Kodamatani et al., 2005; Friedman, 2006). According to Milner et al. (2011), the toxicity of glycoalkaloids could be due to their anticholinesterase activity and disruption of cell membranes, which causes neurological disorders and gastrointestinal disturbances, respectively. The estimated highest safe level, a level that may cause acute toxicity and the lethal dose of total glycoalkaloids for human consumption are about 1, 1.75, and 3-6 mg kg-1 body weight, respectively (Friedman and McDonald, 1999).
In spite of their toxicity, studies also suggest that glycoalkaloids may also possess anti-carcinogenic effects, depending on their dose and conditions of use (Friedman, 2006). Friedman et al. (2005) and Friedman (2015) reported the in vitro and in vivo concentration-dependent anti-carcinogenic effects of α-chaconine and α-solanine from potatoes against the human liver, cervical, lymphoma, and stomach cancer cells.
Therefore, to obtain the optimum benefit of glycoalkaloids and to reduce their potential toxicity to human beings, it is a must to establish guidelines that limit the glycoalkaloid content of new cultivars before releasing them for commercial use (Valcarcel et al., 2014). Also, it is crucial to managing postharvest light exposure, mechanical injury, and storage conditions (Friedman, 2006). Proper storage environment should reduce moisture losses, prevent spoilage by pathogens, and reduce quality deterioration and sprout growth. Although cold storage may prevent loss of weight, spoilage, and sprouting, it can cause freezing injuries, which result in soft and unusable tubers (Chourasia and Goswami, 2001; Nourian et al., 2003). Chuda et al. (2003) also indicated that formation of acrylamide, a possible carcinogen (Tareke et al., 2002), was higher when potato chips were made from the tubers stored at a 2°C (Yoshihiro et al., 2003) as it accelerates the conversion of starch in to reducing sugars. Stadler et al. (2002) revealed that the thermal treatment of specific amino acids in combination with reducing sugars could result in the formation of acrylamide. On the other hand, higher storage temperatures result in rapid quality loss and increased respiratory activity and hence shorten shelf life (De Freitas et al., 2012).
Keeping in view the importance of storage, it is necessary to identify the changes related to the prolonged storage of the potato tubers at different temperatures. Hence, ‘Atlantic’ and ‘Solbong’ potato cultivars were stored for 12 weeks under 10 and 20°C with 85-90% RH, in darkness with the objectives (1) to identify the effects of storage temperature and storage duration on the processing related quality parameters and total glycoalkaloid content of the tubers and, (2) to examine the color changes, acrylamide formation, and overall sensory responses of the potato chips after processing of the stored tubers.
Materials and Methods
Plant material
‘Atlantic’ and ‘Solbong’, the most widely used cultivars for potato chips processing in Korea, were used for this study. Tubers of the two cultivars were obtained from Haitai-Calbee snack factory, Korea, and cured for one week at 15°C in darkness. After curing, each potato cultivar was carefully selected for its freedom from defects, and relatively uniform size potato tubers were then subsequently stored in the dark at 10°C and 20°C with 85-90% RH. Sub-sampling was done at every three weeks’ interval and continued up to the 12th week of storage.
Firmness, fresh weight loss and respiration rate
Firmness measurements were taken at the center of tubers by Sun Rheo Meter Compac-100II (Sun Scientific Co. Ltd., USA) as described by Tilahun et al. (2017) with a maximum force of 10 kg and a 3 mm diameter round probe having a sharp end.
Potatoes were weighed before storage and weighed again at the end of each storage period, and percentage (%) fresh weight loss was calculated as described by Tilahun et al. (2018).
Respiration rates of potatoes were measured as a function CO2 concentration by using the closed system method. Sample tubers were weighed and placed in airtight 4 L volume container for 3 h and CO2 concentration was analyzed using PBI Dan-sensor (Check mate 9900, Denmark) gas analyzer as stated by Belew et al. (2016) and Tilahun et al. (2019). The result was expressed as mL CO2 kg-1 hr-1.
Specific gravity (SG), dry matter (DM) and starch content
Ten healthy and marketable size grade tubers from each treatment were cleaned and weighed in both air (W1) and water (W2), as stated by Vasanthan et al. (1999). The SG was then calculated as W1/ (W1-W2). The method indicated by Kumar et al. (2005) was used to obtain DM. Strip samples were prepared from ten tubers of each treatment subsequently weighed and put in a forced-air oven to dry for 72 hours at 70°C. After drying, the samples were weighed separately. The ratio between dry and fresh mass was used to calculate DM and expressed as a percentage.
$$\mathrm{DM}\;(\%)\;=\;\mathrm{Dry}\;\mathrm{weight}/\mathrm{Fresh}\;\mathrm{weight}\;\ast\;100$$ | (1) |
Starch content in percent was calculated from the measured SG and DM of tubers using the following methods established previously, and the average values obtained from the following equations were used for comparisons.
$$\mathrm{Starch}\;\mathrm{content}\;(\%)\;=\;17.565\;+\;199.07\;(\mathrm{specific}\;\mathrm{gravity}\;-1.0988)$$ | (2) |
(Von Schéele equation cited by Abebe et al., 2013)
$$\mathrm{Starch}\;\mathrm{content}\;(\%)\;=\;17.55\;+\;0.891\;\ast\;(\mathrm{DM}\;(\%)\;-\;24.182)$$ | (3) |
Extraction and quantification of glycoalkaloids
The extraction of glycoalkaloids was done from ten potato tubers for each storage temperature and cultivar at 3 weeks interval during 12 weeks storage period. The samples were not peeled because glycoalkaloids are mainly found in the potato skin or close the skin (Kirui et al., 2009). A 0.5 g of homogenized sample was extracted in 10 mL of chloroform: methanol: water (2:5:2, v/v/v). Extraction was completed by shaking for 10 minutes before centrifugation at 6000 × g for 10 min to remove solids. The clear supernatant was filtered by a 0.22 μm syringe filter. Glycoalkaloids were analyzed using ultra-performance liquid chromatography-tandem mass spectrometry (UPLC-MS/MS (Zywicki et al., 2005).
The UPLC-MS/MS system consisted of Acquity UPLC System (Waters, Milford, MA, USA) and Quattro Premier Tandem Mass Spectrometer (Micromass, Manchester, UK). The analytical column was Acquity UPLC BEH C18 column (2.1 × 100 mm, 1.7 μm particle size) from Waters (Milford, MA, USA). The mobile phase was 0.1% formic acid in acetonitrile: water (40:60, v/v). The injection volume was 5 µL, and the flow rate was 0.4 mL/min. The temperature of the column was kept at ambient temperature. Positively charged ions were detected by mass spectrometric detection using the very selective MRM mode. The mass spectrometer was adjusted as the following: Nitrogen was used as desolvation gas (800 L/h, 350°C) and as cone gas (50 L/ h). Argon was used as collision gas (4.26 × 103 bar). The source temperature was maintained at 120°C, and the capillary voltage was 3.5 kV. For quantification of α-solanine and α-chaconine, the most abundant transitions were used with m/z 868→398 and m/z 852→706, respectively. To have further control of compound identity in potato extracts, the transitions m/z 868→706 and m/z 852→398 were additionally recorded for both compounds. The collision energy was set to 70 eV for both α-solanine and α-chaconine (Matsuda et al., 2004; Nie et al., 2018; Zywicki et al., 2005).
Chipping of the tubers
Tubers were chipped immediately after curing and after storage up to 12th week at 3 weeks interval using Haitai-Calbee Industry, Korea, standard chip processing procedures. Ten tubers from each treatment were cleaned and then sliced separately into 1.5 mm sections. After slicing, the slices were rinsed with water for about one minute to remove water-soluble sugars present on the surface. Consequently, the rinsed slices were placed on strainer trays to remove excess water and fried in soybean oil at 180°C for 2 min and 15 secs in an electrical fryer (DAESHIN Electric Co., Ltd, model-DS 100, Incheon, Korea). The fried chips were drained over a wire screen for 5 min and placed on a plate having a paper towel to absorb excess oil and allowed to cool for 10-15 min. After cooling, the chips were labeled, bagged, and stored at either -20°C for further analysis or at 4°C for sensory evaluation.
Extraction and quantification acrylamide from the chips
Acrylamide content of the chipped samples of potato tubers from each storage temperature was measured with three replications for both cultivars during the storage period. The homogenized sample was weighed 1 g into a graduated 50 mL polypropylene conical tube with a cap. Then, 2 mL of internal standard solution was added (13C3 labeled acrylamide in methanol, 100 µg/ L) followed by 18 mL of water to briefly disperse the test portion in water. The added portions were mixed for 20 min on a shaker before centrifuging at 9000 × g for 20 min to gain a 5 mL portion of clarified aqueous phase for spin filtration and SPE cartridge. Conditioning of Oasis HLB cartridges was done with 3.5 mL of methanol followed by 3.5 mL of water and discarded after conditioning. After loading each cartridge with 1.5 mL of filtered extract, the extract was allowed to pass through the sorbent material, followed by 0.5 mL of water. Then, the column was eluted with 1.5 mL of water, and the eluant was collected for Bond Elut-Accucat SPE cartridge clean up. The A cartridges were conditioned with 2.5 mL of methanol followed by 2.5 mL of water after marking the outside of the Bond Elut-Accucat SPE cartridge at the height of 1 mL of liquid above the sorbent bed. After discarding the methanol and water portions, all of the eluant collected from the Oasis SPE cartridge was loaded and eluted to the 1 mL mark before the remainder of the eluted portions was collected. These portions were passed through a 0.22 μL syringe filter and transferred into 2 mL amber glass autosampler vials UPLC-MS/MS analysis. The mobile phase was 0.1% acetic acid and 0.5% methanol in water. The injection volume was 10 µL, and the flow rate was 0.3 mL/min. The temperature of the column was kept at ambient temperature. Mass spectrometric detection of positively charged ions was performed using the very selective MRM mode, for which the mass spectrometer was set to the following tune parameters: Nitrogen was used as desolvation gas (800 L/h, 350°C) and as cone gas (50 L/h). Argon was used as collision gas (4.63 × 103 mbar). The source temperature was maintained at 120°C, the capillary voltage at 3.5 kV, the cone voltage at 23 V. For quantification of acrylamide, the most abundant transitions were used with m/z 72 →55 and 27 at 9 V. The transition monitored for labeled acrylamide was m/z 75 → 58 at 11 V (Roach et al., 2003).
Determination of color of chips
CR-400 chroma meter (Minolta, Tokyo, Japan) was used to determine Hunter’s L* (brightness) values (McGuire, 1992). Values were measured three times from each piece of ten sample chips obtained from every treatment, and the average was recorded.
Overall sensory evaluation
Sensory evaluations of the sample chips from each treatment was carried out with five trained panelists. The overall sensory evaluation was done as the mean value of the subjective scale of appearance, texture, and taste as described by Seo et al. (2018) on a 9-point hedonic scale (1=disliked extremely, 9=liked extremely). Each sample was assigned code and randomly served with small tray.
Statistical analysis
The experiment was conducted in a completely randomized design with ten replicates for all parameters. The data were subjected to analysis of variance (ANOVA) to determine the significance of differences between cultivars and harvest time during cold storage at p < 0.05 using SAS statistical software (SAS/STAT ® 9.1; SAS Institute Inc., Cary, NC, USA).
Results and Discussion
Firmness, weight loss and respiration rate
Potato tuber texture is a crucial quality determinant that influences consumer’s preferences (Vreugdenhil et al., 2011). Fig. 1A shows the significant (p < 0.05) interactions observed between varieties and storage temperature on the firmness of tubers throughout the storage period. In general, firmness decreased as the storage period proceeded at both 10 and 20°C, and higher temperature resulted in a faster rate of change. At the beginning of storage, the firmnesses were 16.62 and 14.52 N for ‘Atlantic’ and ‘Solbong’ tubers, respectively. The firmness of both cultivars stored at 20°C showed a decreasing trend as the storage period proceeded and reached 10.2 and 11.62 N after 12 weeks of storage for ‘Atlantic’ and ‘Solbong,’ respectively. Meanwhile, the firmnesses of tubers stored at 10°C were reduced slightly and attained 13.58 and 13.54 N after 12 weeks of storage for ‘Atlantic’ and ‘Solbong,’ respectively. Our result complies with the previous work by Nourian et al. (2003), who reported the delayed changes in firmness at lower (4-8°C) storage temperatures.
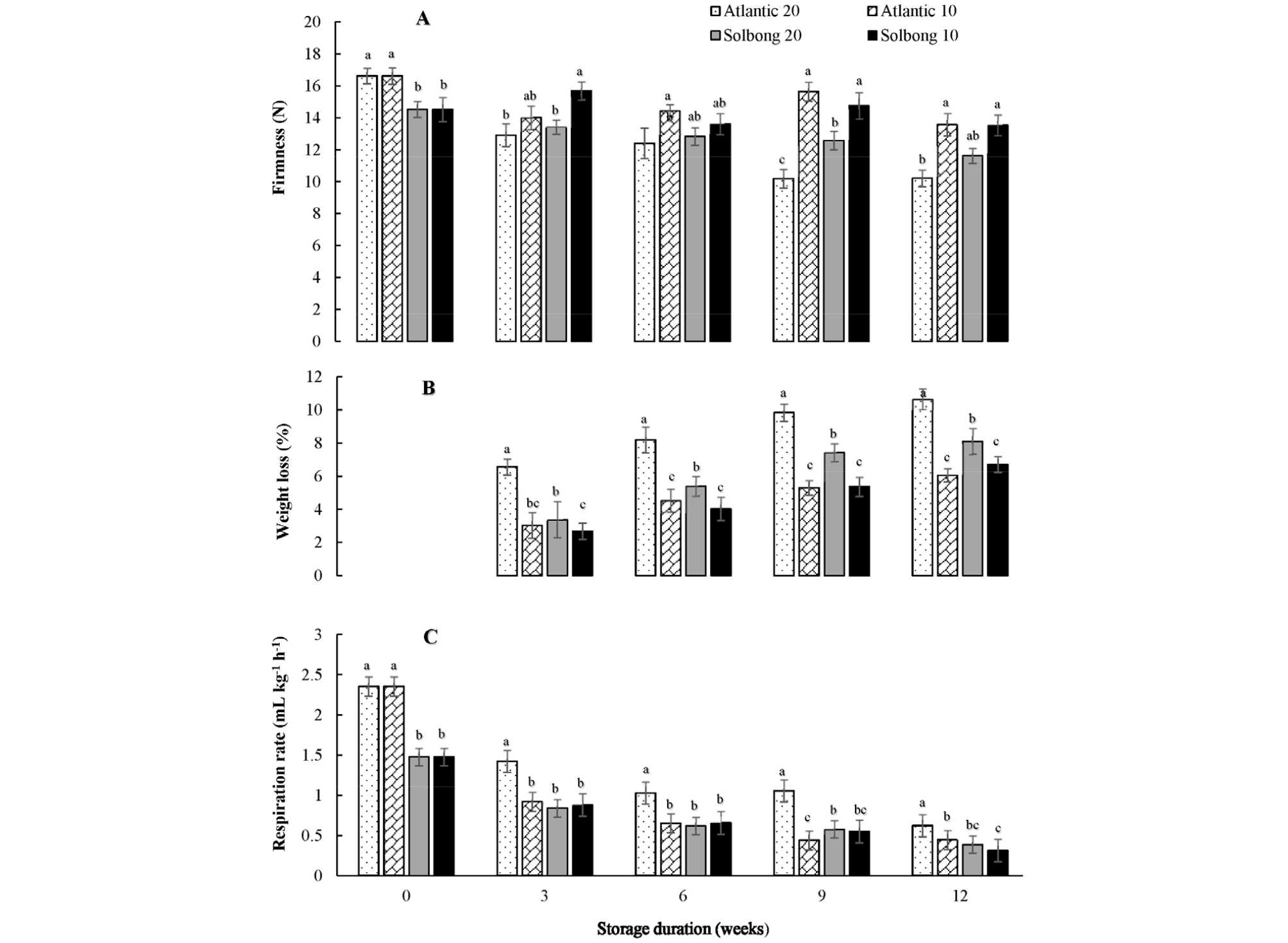
Fig. 1.
Firmness (A), weight loss (B) and respiration rate (C) of ‘Atlantic’ and ‘Solbong’ potato tubers stored at 10°C and 20°C affected by storage duration of up to 12 weeks. Each data point is the mean of ten sample replicates ± standard error. Means with the same letters are not significantly different at p < 0.05.
Potato tuber quality management aims to limit weight loss during storage (Alamar et al., 2017). In this study, significant (p < 0.05) interactions between the varieties and storage temperatures on weight loss were observed starting from the 3rd week up to the 12th week of storage (Fig. 1B). The percentage weight loss of tubers stored at 20°C was higher than tubers stored at 10°C throughout the storage period, irrespective of the cultivar used. Besides, ‘Atlantic’ tubers stored at 20°C exhibited the highest percentage weight loss while both cultivars stored at 10°C were statistically at par. Similar to our present results, significant weight-loss differences were previously reported by Broćić et al. (2016). The weight loss may affect the frying quality, and it could be a reason for the lowest Hunter’s L* value of chips made from ‘Atlantic’ tubers after the 9th week of storage.
Significant (p < 0.05) interactions between verities and storage temperatures on the respiration rate were observed throughout the storage period. The highest respiration rates were recorded at the beginning of the storage and reduced afterward, regardless of the cultivar used. The high respiration rate at the beginning of storage might be an indication of the continuity of the healing process that started during curing right before storage. The respiration rates in this study ranges 0.45-2.35 and 0.31-1.48 mL kg-1 hr-1 for ‘Atlantic’ and ‘Solbong’, respectively. In line with our current work, Schippers (1977) gathered from literature that respiration rates of potato tubers were influenced by cultivar, temperature, and storage duration.
Specific gravity, dry matter, and starch content
SG can be used for estimating DM and the processing quality of potato varieties (Abebe et al., 2013). In this study, interactions between the varieties and storage temperature on SG were significant (p < 0.05) only on the 6th week of storage. However, significant (p < 0.05) interactions on DM and starch content were observed from the 6th to 12th week of storage (Fig. 2 A, B and C). Tuber specific gravity values were categorized as low (< 1.077), intermediate (1.077-1.086), and high (> 1.086) by Fitzpatrick et al. (1969). Tubers having high SG are better suited for baking, frying, mashing, and chipping (Haase, 2003; Pedreschi and Moyano, 2005). Similarly, the report by Kabira and Berga (2003) showed that tubers with a DM of 20 to 24% and SG more than 1.080 are suitable for making French fries. Moreover, tubers having a starch content of 13% and above (Abebe et al., 2013) fulfill the global quality standard. The results of this study revealed that both cultivars under each storage condition showed SG greater than 1.08, DM higher than 24.09%, and starch content above 15.88%. The increase or maintenance in tuber DM and starch content during storage presumably suggested that the relative losses of DM and starch caused by respiration were lower than relative losses of water caused by transpiration. In general, both ‘Atlantic’ and ‘Solbong’ cultivars stored at 10 and 20°C with 85-90% RH were suitable for processing and fulfilled the global quality standards up to 12 weeks of storage in terms of SG, DM, and starch content.
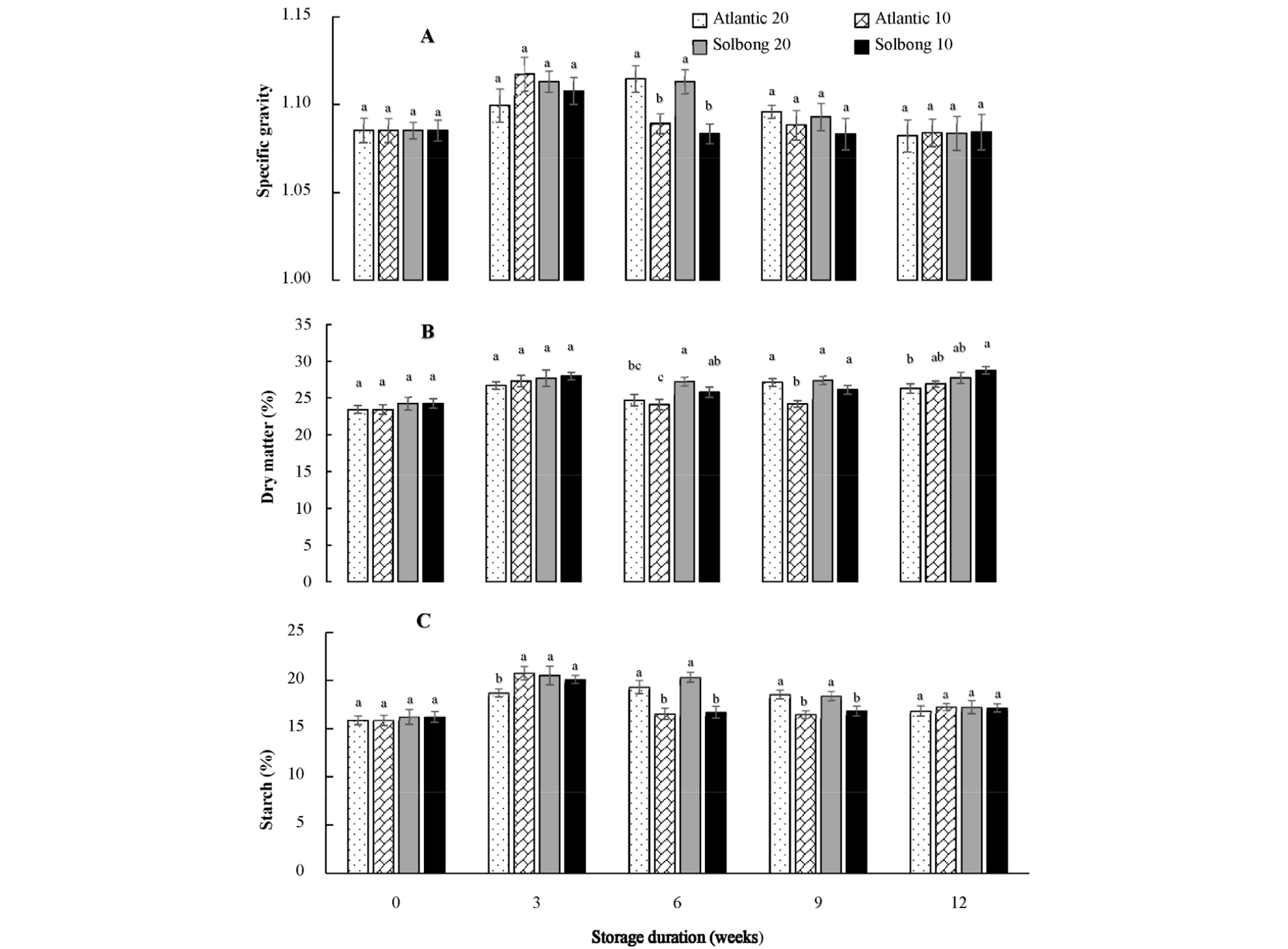
Fig. 2.
Specific gravity (A), dry matter (%) (B) and starch content (%) (C) of ‘Atlantic’ and ‘Solbong’ potato cultivars stored at 10°C and 20°C affected by storage duration of up to 12 weeks. Each data point is the mean of ten sample replicates ± standard error. Means with the same letters are not significantly different at p < 0.05.
α-solanine and α-chaconine and total glycoalkaloids
The glycoalkaloid contents of both ‘Atlantic’ and ‘Solbong’ cultivars in the present study were significantly (p < 0.05) affected by storage temperature throughout the storage period (Fig. 3 A, B and C). ‘Solbong’ stored at 20°C had the highest α-solanine, α-chaconine, and total glycoalkaloids, followed by ‘Solbong’ stored at 10°C, throughout the storage period. On the other hand, the lowest glycoalkaloids contents throughout the storage period were recorded from ‘Atlantic’ stored at 10°C. The overall results showed that the effects of storage on glycoalkaloids content were cultivar dependent in agreement with various previous works (Kumar et al. 2004; Bejarano et al., 2000; Cantwell, 1996). Also, our results revealed that storing potatoes at lower a temperature (10°C) kept the glycoalkaloid content of both cultivars lower than storage at a higher temperature (20°C), throughout the storage period. Similarly, Cantwell (1996) stated that storing potatoes at low temperature and keeping the tubers away from light keep the glycoalkaloids content low. In this study, the total glycoalkaloid content of ‘Atlantic’ and ‘Solbong’ at the beginning of the storage were 59. 41 and 84.54 mg kg-1, respectively. After a progressive increase, the total glycoalkaloid content reached 102.71 and 92.31 mg kg-1 for ‘Atlantic’ stored at 20 and 10°C, respectively, after 12 weeks storage. Meanwhile, 189.06 and 144.89 mg kg-1 were recorded after 12 weeks of storage for ‘Solbong’ stored at 20 and 10°C, respectively (Fig. 3C). An upper limit for glycoalkaloids content of 200 mg kg-1 of potato is generally accepted for food safety purposes (Friedman, 2006; Kodamatani et al., 2005; Sotelo and Serrano, 2000; Cantwell, 1996; Coxon et al., 1979). Hence, based on glycoalkaloids content, ‘Atlantic’ and ‘Solbong’ stored at 10 and 20°C with 85-90% RH were safe for chips making up to 12 weeks of storage.
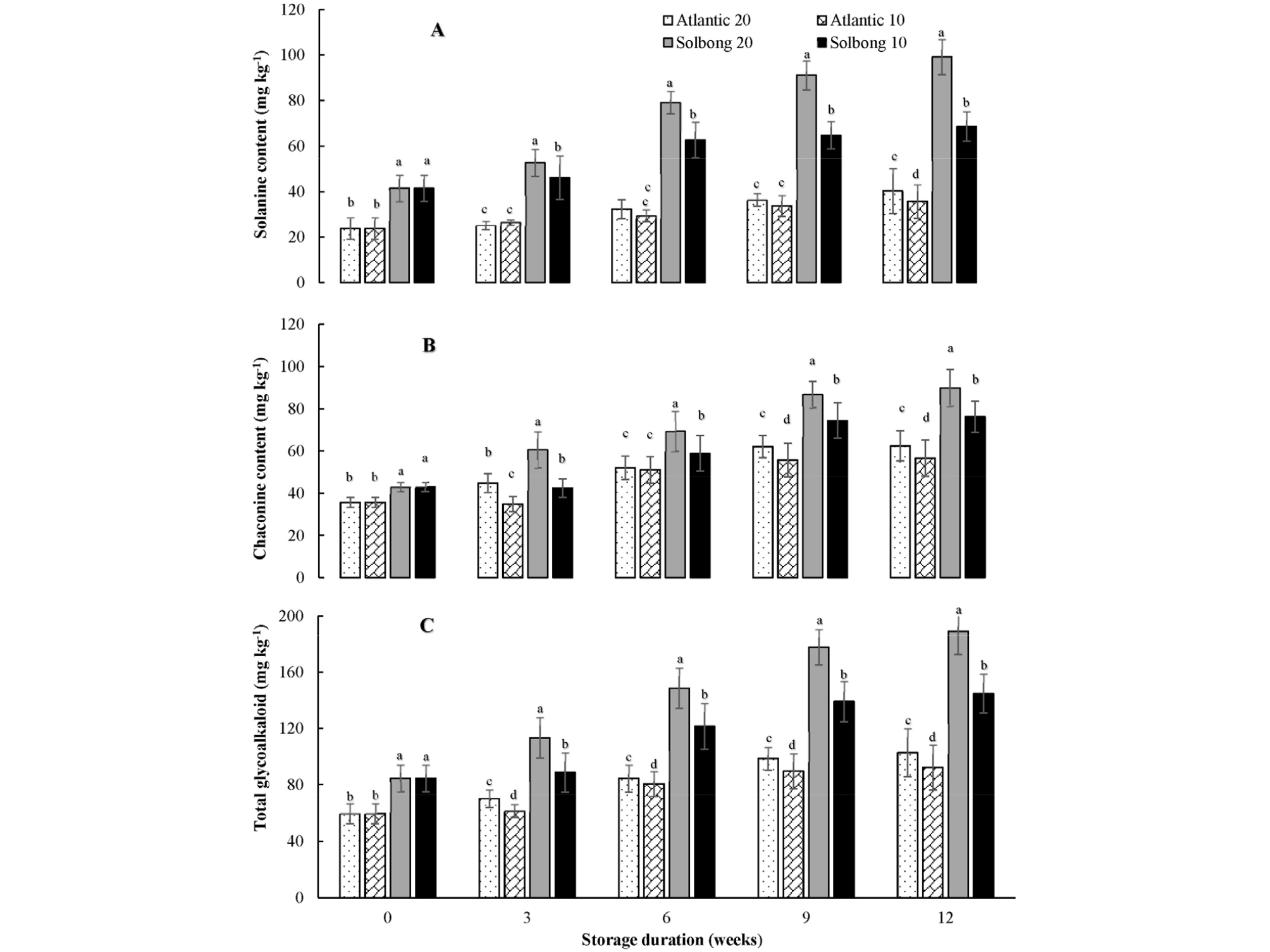
Fig. 3.
α-solanine (A), α-chaconine (B) and total glycoalkaloid content (C) of ‘Atlantic’ and ‘Solbong’ potato tubers stored at 10°C and 20°C affected by storage duration of up to 12 weeks. Each data point is the mean of ten sample replicates ± standard error. Means with the same letters are not significantly different at p < 0.05.
Acrylamide content, color changes and sensory evaluation of the potato chips
Interaction between cultivars and storage temperatures on acrylamide formation was significant (p < 0.05) throughout the storage period. ‘Solbong’ tubers that stored at 10°C appeared to be more suitable for chips production in terms of acrylamide formation as there is the lower formation of acrylamide compared to ‘Atlantic’ tubers stored at the same condition. Our results are similar to the previous report of De Meulenaer et al. (2008), which suggested that the acrylamide content of potato chips is cultivar dependent. Storing potato tubers at 20°C seemed to enhance acrylamide formation as the storage period proceeded especially in ‘Atlantic’ cultivar (Fig. 4A). Kumar et al. (2004) indicated that the storage of potato tubers at 20°C or higher temperatures could lead to an increase in sugar concentration. Similarly, Nourian et al. (2003) also indicated more rapid changes in different quality parameters of tubers at 20°C. The changes in quality of tubers due to storage at higher temperature may favor the formation of acrylamide from asparagine and reducing sugars by Maillard reaction during frying of the potato chips (Bethke and Bussan, 2013). Yoshihiro et al. (2003) indicated the temperature range (8-12°C) for the storage of potato tubers to prevent deterioration of chips, which has direct correlation with acrylamide formation. Tolerable daily intake levels of acrylamide were reported to be 40 and 2.6 µg/kg/day for neurotoxicity and cancer, respectively (Tardiff et al., 2010). The results of our research underlined the fact that consumers should consider their daily intake of potato chips based on their body weight for safe dietary intake as it is a considerable contributor to acrylamide. Katz et al. (2012) and Elmore et al. (2015) also indicated that substantial proportions of acrylamide in daily consumption were contributed by cooked potato products like crisps, chips, and oven cooked potatoes.
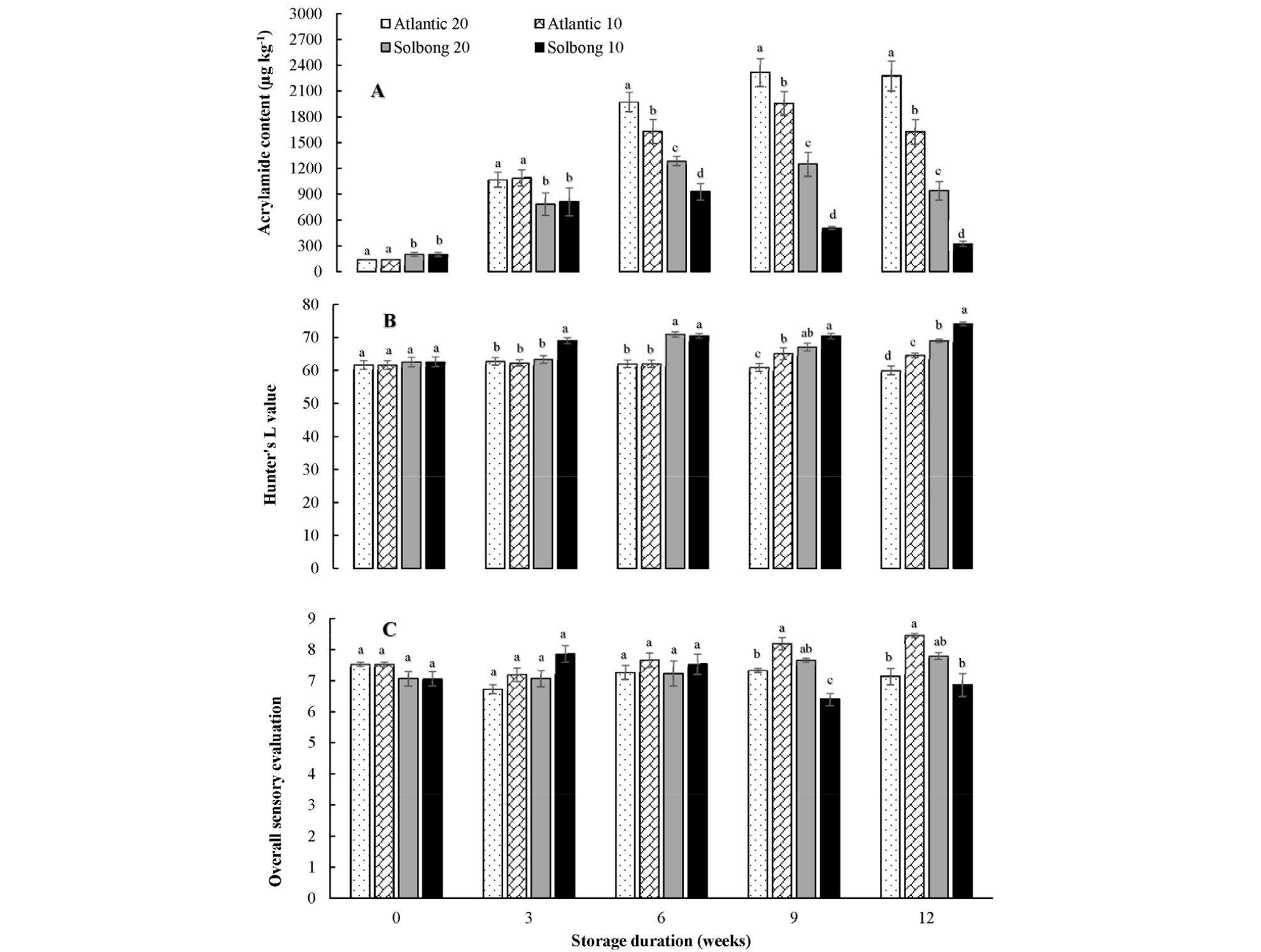
Fig. 4.
Acrylamide content (A), Hunter’s L value (B) and overall sensory evaluation (C) of the chips of ‘Atlantic’ and ‘Solbong’ potato tubers stored at 10°C and 20°C affected by storage duration of up to 12 weeks. Each data point is the mean of ten sample replicates ± standard error. Means with the same letters are not significantly different at p < 0.05.
Conclusions
Our study revealed that both ‘Atlantic’ and ‘Solbong’ cultivars have high specific gravity and dry matter content ,and could be suitable for extended storage without significant deterioration in processing quality. However, significant interactions between the cultivars and the storage temperatures were observed in influencing the storage quality of the tubers and affected the quality of potato chips. Generally, ‘Solbong’ tubers stored at both 10 and 20°C were found to be better stored with a high dry matter and starch content. Interestingly, ‘Solbong’ tubers stored at 10°C were also suited to produce chips having a brighter color and low acrylamide formation. Also, there was a progressive increase in total glycoalkaloids of both cultivars during the storage period, particularly at 20°C. Still, it did not exceed the safe upper limit of the glycoalkaloid content of the tubers for human consumption. Nevertheless, the tubers of both cultivars stored at 10°C were suitable for processing without the formation of a high level of α-solanine and α-chaconine until 12 weeks. Besides, the acrylamide formation of both cultivars was kept low by storage at 10°C. Hence, storing tubers of both cultivars under 10°C with 85-90% RH, in darkness, could prolong the shelf life of tubers without affecting the nutritional quality during processing.